Ultrasound
The use of ultrasound in various forms of measurement has been with us for over 40 years. Today, the use of portable ultrasonic equipment is becoming the standard tool to use for finding airborne leaks, testing steam traps, finding corona discharge, bearing condition testing and tightness testing. But how does this type of ultrasonic measurement work?
Ultrasound is defined as noise beyond the range of human hearing. For the application to airborne leak detection, the frequency at which the most noise is produced by a turbulent leak is 38.4kHz. So, produce an instrument which listens to this frequency and you can detect leaks - but the human can still not hear them. To overcome this problem some electronic processing is required.
The type of electronic processing of the data used varies slightly by manufacturer. This work was performed using an SDT International model 170 which, for the vibration-literate, uses a demodulation method to extract sum and difference frequencies.
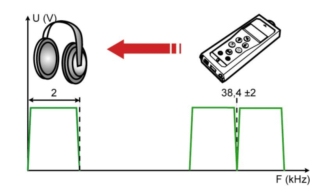
The processor in the SDT170 generates a 2kHz filter above and below the operating centre frequency and takes the sum and difference frequencies to produce a new signal with a 2kHz bandwidth which can be heard - and measured. Using this method it is possible to listen to ultrasounds. By changing the sensor arrangement connected to that instrument, it becomes possible to listen to ultrasounds generated by different mechanisms.
The beauty of this technique is that the audio signal is a reasonable analogue of the original rather than a series of Geiger-counter clicks or buzzes. From an analytical viewpoint, the beauty of this method is that there is a signal which contains real and useful data.
Fault Finding
Using an airborne ultrasound system it is possible to walk around even the noisiest of environments and listen for ultrasonic defect signatures. The reason why, is simple. The normally high levels of noise that we are used to dealing with in the plant is almost entirely noise generated solely in the audio region - very little of the normal noise contains ultrasonic components.
Taking a walk around motors, bearings, belts, gearboxes, pumps, vacuum lines, steam traps and compressed air lines will take on a whole new meaning when listening ultrasonically. Motors with electrical problems will crackle as you listen to the corona, bearings will have a smooth rushing sound if they are OK and will have a popping sound if there is a problem. Belts, gearboxes and pumps will squeal. Pumps will also pop if they are cavitating. Perhaps some of the most used applications of ultrasound are airborne leaks and steam trap testing.
Energy Efficiency
The sad fact is that industry wastes Millions each and every year. That this loss is almost entirely controllable and is ultimately controlled by human error has still to sink in.
Using a portable ultrasound system it is possible to find not only compressed air leaks but also vacuum, steam and nitrogen leaks, as well as some refrigerant gases.
Changing from an airborne sensor to a contacting ultrasound probe and you have the perfect tool for listening to steam traps. Once again it does not matter how noisy the environment is in terms of audible noise, the ultrasound system picks up the operation or otherwise of the steam trap.
Lubrication improvement
The same contact probe can be used to listen to bearing condition. By attaching the contact probe to a modified grea
It is becoming increasingly apparent that lubrication must move from time-based and often infrequent greasing to a comprehensive on-condition approach. This method will prevent not only under lubrication but also the equally deadly over lubrication which is so prevalent in many plants.
A simple and common approach to monitoring and setting baseline friction levels is with an ultrasonic data collector. Friction from the bearing excites the sensor which in turn produces a microvolt signal which is accurately measured and referenced to a decibel log scale. This data is displayed on-screen and stored to the internal data collector. It can then be transferred to PC where for further analysis. As a rule any bearing with a measured ultrasound value 8-10 dBµV over its baseline should be greased using this method.
If an ultrasound system can do all of this, the next logical step must be to consider integrating ultrasound and vibration: to use an ultrasound system as an intelligent sensor for a vibration system. But first there are some basic problems to overcome.
Repeatability
Coming from the world of vibration, we are familiar to one degree or another with the concept of repeatability of our measurement. Sometimes we use accelerometers with vaguely known sensitivities in instruments which are poorly calibrated and then claim a change of 10% in a reading to be significant. This is perhaps an extreme case of bad measurement method, but in the world of ultrasound there are other problems to overcome in terms of repeatability. The greatest problem is to answer the question "What exactly am I measuring?".
In High School science experiments, clearly stating the measurement units was an essential part of good lab technique. Yet, in the world of ultrasound this most fundamental point has been almost completely ignored.
Since ultrasound is an acoustic measurement, it seems quite logical to use the global acoustic unit of amplitude, the decibel or dB. But, the dB has no meaning unless it is quoted against a reference. Sadly within the airborne ultrasound world this basic fact has been overlooked by many of the manufacturers. It is for this reason that this work was carried out using the SDT170 because the SDT unit is measured in dBµV, the sensors are calibrated, traceable and have proven repeatability.
Again, in our vibration world, we take it for granted that if I have 3 accelerometers and 3 data collectors I can use any of these 9 combinations to take a reading on a machine and get the same result within a small statistical spread with some repeatability. This has been shown not to be so easy to do with some of the ultrasound systems on the market. Though this is of course essential if we are to deploy ultrasound into the trending and diagnostics world inhabited by the vibration engineer.
What does an Ultrasound signal show?
It is one thing to go around listening to signals, but is it possible to use this technology for measurement and for diagnosis?
Here we see an example of an ultrasound signal produced by a contact probe and captured by a data collector.
Here is the same bearing captured with an airborne probe:
There is one clear aspect to these two signals: they are impulsive in nature, not sinusoidal nor repetitive, but consisting of a series of sharp pulses.
This does not bode well for someone planning to use FFT processing on these signals. Take a look at this comparison of the corresponding spectra for the two signal above:
Ignoring the vertical axes and the absolute numbers, it is clear to see that there is little difference between these two. How wrong could we be?
The reason for this error goes right back to the basic mathematics of the FFT. Basically the FFT is only supposed to work on a repetitive signal from -∞to ∞. Outside this basic condition, the result may not be as good as you think.
The classic time signal for a bearing defect gives a goldfish envelope like this:
The spectrum however only really shows the resonance, why because that is where all of the repetitive energy is in the signal.
So, relying on spectrum analysis of ultrasound signals in the case of the transient signals associated with bearings is not necessarily a good idea. Spectrum measurement works much better when there is a much lower peak-to-mean ratio. Take for example in the area of corona and discharge. Here is a comparison of two time signals, one is nuisance corona and the other is a damaging corona:
Here we see that in the case of the nuisance signal on the bottom, the peak-to-mean ratio is quite low and that this ratio does decrease slightly as the defect deteriorates. In a case like this there is some safety in applying FFT analysis. In doing so we get the following:
In the nuisance signal we see a series of harmonics of 120Hz but in the damaging signal we see a series of harmonics of 60Hz. So in this case we can use all of the standard methods we use in vibration analysis to assist in a simple detection method for damaging corona.
Conclusions
1. Storing the signal generated by an ultrasound system can be beneficial
2. The ability to visualise a transient event and characterise it is helpful in the analysis and diagnostic process
3. It is important to select the recording process appropriate for the diagnosis you wish to perform
4. Storing data in a structured manner inside a predictive maintenance database program with the analysis tools that provides will open up new application areas
5. Airborne ultrasound alone can pick up defective bearings
6. Great care must be taken when using spectrum analysis on signals with large peaks and low energy since the spectrum calculation process can mask or destroy the pertinent information
7. Any proprietary predictive maintenance system selected should include functions which allow the importing, manipulation and post-processing the time signal
8. Any future developments which would provide the raw broadband ultrasound signal would mean that the user could apply other standard DSP analysis tools to the recorded signal.
Biography
Tom Murphy is an Acoustics graduate from Salford University and has 25 years experience in the world of industrial vibration measurement.
Tom is the Managing Director of Adash 3TP Limited, based in Manchester England, a Company specialising in the application of vibration, infrared and ultrasonic technologies to improve maintenance.